Road Bike Crank Stiffness Test
- Posted on
- By Steve, Garrett, and Jason
- Posted in Tech
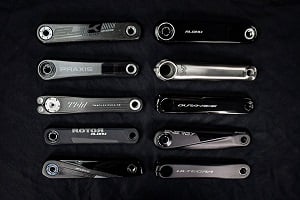
In celebration of Fair Wheel Bikes' 50th Anniversary we have dusted off our stiffness testing apparatus to once again measure crank stiffness. This time we have a pile of modern road bike cranksets to see where each crank manufacturer stacks up.
As a lot of you tuning in already know, we’ve been performing on our in-house road crank tests for more than a decade.
Luckily, Jason Krantz is back as our resident data-cruncher (Jason is an experienced mechanical engineer with a strong background in both finite element analysis and composite materials.) We’re always happy to have him work with us on our tests and place a high value on his contributions.
Disclaimer: A lot of typing and numbers have gone into this article and we apologize in advance for any typos, but would warn that the possibility of mistakes is present.
About the testing method: Each arm was preloaded with 50 lbs of weight, then all calipers and load cells were zeroed out. Another 150 lbs was added and the difference was measured in mm. Each arm was tested three times and an average of those measurements is the result. A lower number represents a stiffer crank. These will be labeled as Deflection-D (Drive side deflection) and Deflection-ND (non-drive side deflection). This method uses a total load of 200 pounds.
Stiffness/Weight: Is determined by: (1/average deflection)/weight)x10000
Notes about stiffness: According to conventional wisdom, the more pedaling stiffness the better. Stiffness implies efficiency, along with the notion of a stiffer bike inspiring confident and responsive handling. As desirable as those traits are, and even after a crank’s stiffness is proven empirically, the outcome still seems subjective—we are simply going by how many people feel more efficient on a bike with a stiff drivetrain. But it’s far from clear whether that tactile, from-my-quads-directly-to-the-road sensation is actually faster. This is the question: is a stiffer drivetrain actually more efficient, or does it just feel that way? Looking at the numbers, we can see that average deflections range from roughly 5mm to 8mm. From this, we can generalize and say that the most flexible crank is about 50% more flexible than the stiffest crank. It’s easy to imagine that the stiffer cranks feel better, or have better “power transfer,” which is a particularly vague and ill-defined concept.
So how can we move beyond “feel” and attempt to quantify whether a stiffer crank is better? The answer is, strain energy. Strain energy is simply the energy stored by an object as it is loaded. Quantifying how much energy is stored by a given spring under a particular load is a basic problem that works perfectly well for understanding whether a stiff bicycle crank is better than a slightly less stiff crank. That is, you can think of a bicycle crank as a very stiff, oddly-shaped spring. The equations for calculating stored energy in a beam under bending are fairly simple.
A crank is somewhat beam-like, but it’s not really a beam. And we’re not applying a pure moment but rather a force at a distance that creates a moment. This bending example is somewhat close, but it’s still not a very good approximation of a crank on a bicycle. We can get a very good approximation of a crank on a bicycle by using finite element analysis (FEA). To find out how much strain energy a typical crank stores, we can solve an FEA model to find the strain energy of each of the constituent elements. We can then add up all of those strain energies to get the strain energy of the whole crank. This strain energy can then be converted into absorbed power by assuming a cadence; we used 100 RPM for this example. In this way, we can determine exactly how much power goes into crank flex, which can then tell us how much crank flex matters to total power output. We used ANSYS, a well-regarded FEA program, to model a generic aluminum left crankarm (172.5mm) and half an attached 24mm steel bottom bracket spindle. The remote force works out to 250 pounds of force (lbf), and it is applied 60mm to the outside of the center of the pedal threads. This simulates applying the force through a pedal.
Most of the action is happening on the inside of the crank; the interior elements report a higher strain energy value than the external ones do. But things get a lot more interesting when we dump the strain energy values for each of the elements to a spreadsheet. By summing the elements’ strain energy values, we can get the total strain energy for the entire crank and half BB spindle. The total strain energy of this crank under a 250-lb pedaling load is 4.604 Joules. As a unit, Joules don’t do much for most cyclists. We can convert them to a more useful unit by assuming a cadence of 100 RPM. At that cadence, this half-crank soaks up 7.67 watts. The right-hand crank is usually stiffer than its left-hand counterpart, so it stores correspondingly less strain energy. So rather than doubling the left-hand figure, we’ll round down a bit to 14 watts for the entire crank/BB axle system. 14 watts might sound like a lot, and it is. But let’s keep in mind that this is a 250-lb force applied 1.67 times per second.
A rider applying this force over 160 degrees of crank rotation produces an average power of 880 watts, which few of us can sustain for long. And 14 watts out of 880 is 1.6% To put this in perspective, if you were pedaling along at a steady 300 watts, your crank would be absorbing 4.8 watts of your effort. But those 4.8 watts go into winding up your crank “spring,” which will spring back with nearly all the energy that was spent winding it up.
Some of that spring-back energy probably helps turn the drivetrain while some of it may behave in a negative manner. However, there’s a fair amount of debate about how much energy is returned. The answer to the energy-return question involves kinematic analysis far outside the scope of this article. For now, we’ll assume that all of those 4.8 watts spring back in a way that doesn’t help turn the drivetrain nor hinder it. As mentioned before, the most flexible crank in this review shows about 50% more deflection than the stiffest crank. Our FEA crank is quite flexible, and it absorbs 4.8 watts of a 300-watt effort. Strain energy, roughly speaking, is inversely proportional to stiffness. We can use these relationships to calculate that at 300 watts, our flexible crank absorbs 4.8 watts, or 1.6% of total power output. Meanwhile, a 50% stiffer crank absorbs 3.2 watts, or 1.07%, in strain energy (technically, strain power). That’s a difference of 1.6 watts (or 4.7 watts at our tested 880 watts).
Remember, this assumes that no strain energy is returned to the drivetrain. That’s not to say that crank stiffness is irrelevant, there is a measurable difference. It also provides all the psychological and “feel” benefits described at the beginning of this section. A stiff crank also incrementally improves efficiency by keeping bearings aligned, keeping the pedals more directly beneath your feet, etc. Keep in mind that this 1.6% calculated strain energy absorption represents an upper bound of energy dissipation—that is, the maximum possible energy loss due to crank flexure. Real cranks are much stiffer than our modeled crank and therefore store less strain energy. Moreover, a great deal of that stored strain energy is likely returned to the drivetrain. The real-world losses to crank flexure are, in all likelihood, a fraction of that number.
Things get slightly more complicated for carbon cranks. Composite materials really do damp vibrations better than most metals, and cyclists treasure this property to ease their stinging hands as they hammer over Belgian cobbles and hop curbs on their way to the local coffee shop. But damping is just another word for energy absorption, and a carbon crank certainly absorbs and dissipates more energy than a metallic crank. Modeling that dissipation requires, among other things, intimate knowledge of the crank’s construction (“laminate schedule,” in the argot of composites engineering) But even if we can’t quantify carbon crank losses due to damping, are they worth worrying about? Almost certainly not. They are a tiny addition to the already-small losses in a metallic crank.
Notes about Crank Length: Over the years a lot of different arguments have been made about the benefits of longer/shorter cranks. None of which has really been thoroughly tested until Jim Martins study. Martin showed that length didn’t statistically matter when it came to power, once power was averaged around the entire pedal circle and not just in the forward position, it turns out that shorter cranks (down to 145mm) produced more average power than a longer crank. This conclusion however considers only average power and not other factors which definitely have a bearing on real world use. Damon Rinard followed up the Martin study with some of his own testing comparing the aerodynamic differences in crank length. In almost every case there was an aerodynamic improvement with the shorter crank and without a loss in power. So the power advantage and aerodynamic advantage, combined with shorter cranks generally allowing for a more aggressive or more comfortable position on the bike and less chance of repetitive motion injury, we feel that shorter cranks are something most people should consider. We’re not saying they’re right for everyone, but if you’re on the fence as to which size is best for you, we suggest that you go for the shorter. If you’re interested in more on crank length we suggest reading the above articles.
Notes about weight: Some cranks claimed weights include rings and bb in their complete weights while others do not. To have a level and fair comparison all cranks in this test will be tested and weighed with their stock bsa bottom brackets, and any crank that does not include rings will use our Praxis rings and KCNC alloy chainring bolts.
And with that said, on to the results:
Here's a master list of all the cranks we have tested so far including cranks from years past:
And the winner is: You, the rider. This testing is not done to determine a clear “winner” in the overall best crank category. Every rider, bike, and situation have different sets of requirements and finding a winner would mean defining precisely what requirements you were using. But just as the 100 pound seated climber probably cares little for overall stiffness numbers, the 200 pound sprinter probably cares just as little about overall system weight. Then when you mix in gravel, single ring applications and all the riders in between our two examples, you can see there can be no clear winner. However as previously stated the goal is not to find the best overall crank, but rather to find the best crank for your specific set of requirements.
This crank test will be updated as we gather more cranks and would love to have community participation. Feel free to reach out to us if you want to have your crank tested!